So now we have a solid foundation for what we mean when we speak of failure (an inability to continue the set with technical integrity)…But what are the implications of training like this? What actually happens when a set is taken to failure?
The purpose of resistance training is to elicit specific physiological effects that amount to positive adaptations. And to achieve these effects, a threshold of intensity (I.e. proximity to failure) must be achieved. Typically, the hypertrophic cascades of intra and extracellular signaling begin around 5 reps shy of failure, and increase in magnitude with every rep that closes the gap. This is the crux of the Cult of Intensity’s theory—If proximity to muscular failure equals increased positive adaptations then ipso facto that should also mean that training should always be aligned with this conclusion for best results.
But what is often left out (whether this is intentional or not isn’t for me to opine here) is the cost that comes with this way of training.
While every rep closer to failure will promote a greater stimulus, it will also create corresponding increases in fatigue. If this was a 1:1 relationship, we might be able to turn a blind eye; just sleep a bit more, throw in an epsom salt bath or two, and weather the storm while soaking up all those gains. But my suspicion is that this isn’t the case. It seems much more likely, in fact, that the rep-to-rep hypertrophic potential from approaching failure sees diminishing returns while the accumulation of fatigue is linear if not exponential. This creates a perpetual trade-off—a delicate dance between training hard enough to promote muscle growth while not popping the cork of runaway fatigue.
So despite what some would wish to speak into existence, we can’t have our cake and eat it too.
For the sake of completeness, I want to take a quick technical dive into the low-level physiological and psychological underpinnings that lie at the foundation of the effects of failure training. Feel free to skip this section if you’d prefer to stay high level…
Let us first zoom in on the individual muscle cell:
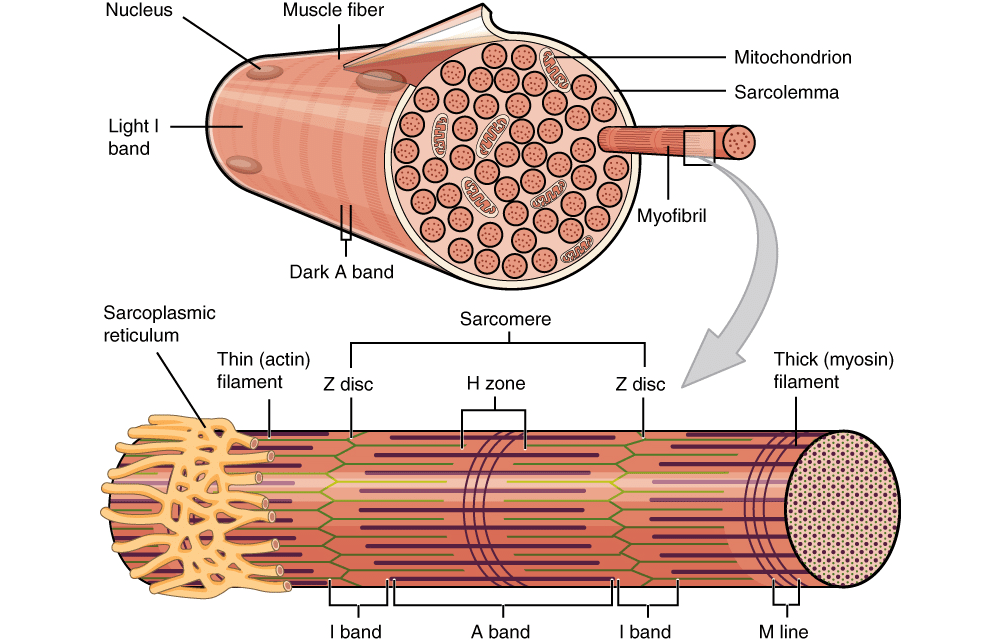
The sarcomere is the basic unit of life-in-motion. It is a microscopic, tug-of-war machine that powers every muscle contraction, from the blink of an eye to the beat of a heart. But this biological machine is made out of fragile protein structures rather than fortified steel; and they are not indestructible. When we purposefully challenge our muscles with resistance training, we expose them to forces that are literally tearing them apart. The ability of our sarcomeres to cope with this damage is what will inevitably lead to growth and adaptation—or atrophy and degradation.
What dictates this ability is a remarkable process that involves stretching, tearing, inflammation and repair. When we perform repeated eccentric contractions and train close to failure, we stretch segments of our muscle fibers beyond their optimal length for producing force. This causes some regions of the sarcomere to bear more tension than others, resulting in micro-tears in the filaments that slide past each other during contraction. These micro-tears trigger an inflammatory response that brings additional blood, swelling and nutrients to the affected area. But they also activate dormant satellite cells, which can fuse with damaged muscle fibers and add new sarcomeres in parallel through the expenditure of ATP (in the form of excess energy/calories) and amino acids (in the form of excess protein). This increases the thickness and density of muscle fibers, which makes them more resistant to future stretching and reduces the risk of injury.
This process of hormesis is one of nature’s ingenious inventions for protecting and propagating the genome—that big and pretty muscles happen to be a particular side effect in mammals is more of a bug than a feature. In an act of biological alchemy, our muscles can turn damage into growth by harnessing the power of their own intrinsic repair mechanisms.
Yet, this seemingly magical process betrays the fine-line of fragility under which our humanity sits atop. We’re dependent on the reliability of our immune response, the availability of the building-blocks of recovery, and the capability of multiple, complex systems to sync perfectly in an environment of relentless dynamism.
The margins between hypertrophy and atrophy are razor thin even in times of surplus. Putting a thumb on the scale through a net caloric deficit, poor sleep, suppressed hormones, and/or overtraining can quickly shift the balance from a state of building, repair, and growth to that of regression, depletion, and withering.
Anatomically, it’s pretty easy to follow along with the sarcomere and conceptualize why more isn’t always better when it comes to training and the intensity in which you train at. But to grasp the full picture, we need to follow a similar path through the brain:
Neurons are the living circuitry of the brain and the literal sparks of life. Their electrical impulses get blasted throughout our central and peripheral nervous systems, coordinating thoughts in our brain, movement in our muscles, and feelings in our viscera. The neuron is the instantiator and orchestrator of our being—yet they are more than just passive conduits of action potentials. These are dynamic and adaptable cells that can change their shape, function, and connections in response to experience.
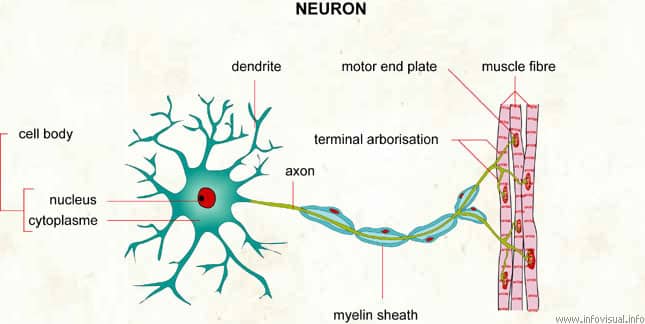
Jumping back to anatomy for a second—a neuron has three main parts: a cell body, an axon and dendrites. The cell body contains the nucleus and other organelles that maintain the cell’s metabolism and gene expression. The axon is a long and thin projection that carries signals away from the cell body to other neurons or target tissues. The dendrites are shorter and branched projections that receive signals from other neurons or sensory input.
The communication between neurons occurs at specialized junctions called synapses. A synapse consists of a presynaptic terminal (the outgoing end of an axon), a postsynaptic terminal (the incoming end of a dendrite or cell body) and a synaptic cleft (the gap between them). When an electrical impulse reaches the presynaptic terminal, it triggers the release of chemical messengers called neurotransmitters into the synaptic cleft. These neurotransmitters bind to receptors on the postsynaptic terminal, causing changes in its membrane potential and influencing its activity.
The brain contains approximately 86 billion neurons. Any given neuron can have up to about 10,000 connections with its neighbors. In case you don’t want to do the math, that is a lot of synaptic potential…And these massive networks enable the brain to process gigabytes of raw information in parallel, integrate and compress the inputs, and create an accurate multi-sensory projection of the outside world. At the heart of this ability to internalize the external is the plasticity of each individual neuron. By consistently modifying their synaptic strength and connectivity in response to learning, memory and/or injury, they allow for adaptation to ever-changing environments and demands.
But what happens if we begin to push this system beyond its optimal environment? How do neurons (and our nervous system as a whole) react and adapt to compounding physical stressors?
To make the case clear, let’s use Overtraining Syndrome as our extreme example. Grossly oversimplified, OTS is a condition that affects athletes who train above their recovery capabilities for too long. We can easily intuit the physical repercussions because they are the same manifestations as acute under-recovery: soreness, fatigue, and decreased performance. But the shift from acute to chronic comes with novel problems. The negative feedback loops retreat inward. The outward, mostly-physical morph into the invisible and mostly-imperceptible. At the extreme ends of overtraining and under-recovery, our brain function is actually suppressed.
The most glaring evidence of this lies in the neurotransmitters that modulate brain activity. Neurotransmitters such as serotonin, dopamine, norepinephrine and acetylcholine pull the strings of our motivation, arousal, attention and reward processes. In OTS, the systems that control the release, motility, reception, and reuptake can become imbalanced due to overstimulation or depletion. The havoc that can be levied when neurotransmitters are out-of-wack is as self-explanatory as it is beyond the scope of this article. But it’s important to understand what all this means at a high-level—that neurotransmitters are how our neurons (and nerves) propagate action potentials, and dysfunction in this context literally means that our nervous systems will not work as intended. For our purposes in this article, the resultant central fatigue—a decline in voluntary motor output due to changes in brain function rather than peripheral factors—can have disastrous effects on performance and, by proxy, hypertrophy.
But it’s not just neurotransmitters that we have to worry about when playing chicken with our nervous system…We also have particularly delicate hormones that react to stress and regulate the subsequent response.
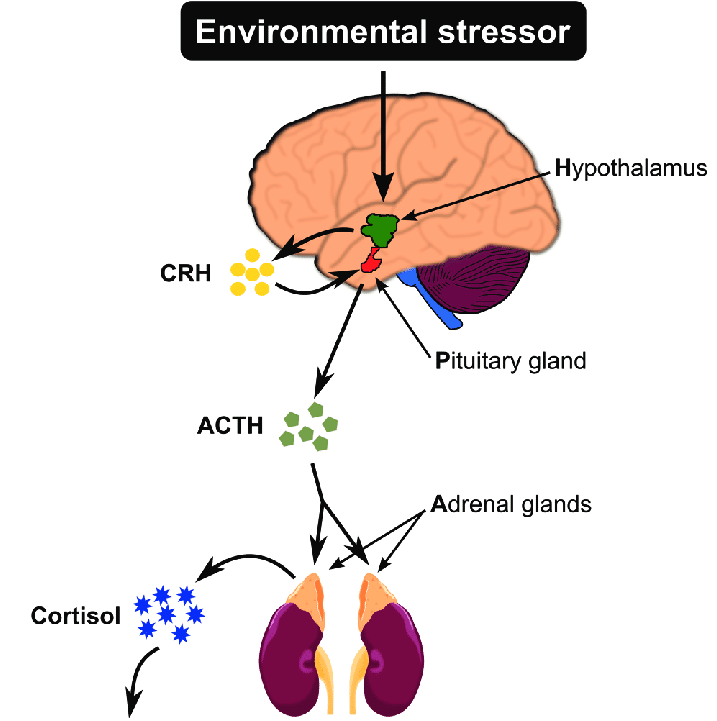
The hypothalamic-pituitary-adrenal (HPA) axis is a network of glands that secrete cortisol, adrenaline, and other hormones that help us manage the challenges of daily life. With normal levels of physical exertion, the HPA axis naturally releases pulses of cortisol and adrenaline as a way to control for the acute bodily stressors and liberate stored energy to repair any damage incurred. However in OTS, the axis becomes overworked and thus less responsive to increasing levels of exercise stimuli, resulting in lower levels of circulating hormones. This pendulum effect may indicate that the body has reached a chronic state of exhaustion and is therefore unable to adapt to environmental stressors. Additionally, we have other players in this glandular hodgepodge that can be negatively affected by our pushing too hard for too long: the HPG axis (hypothalamic-pituitary-gonadal) and the HPT axis (hypothalamic-pituitary-thyroid) see a net suppression of the sex hormones and thyroid hormones, respectively. All in all, this is very bad if we’re at all concerned with being a functional human being, much less on who is big and strong.
Alright, that’s enough of that…Let’s zoom back out and take another look at what these quirks of anatomy and physiology actually mean for training to failure as we care about it…